Polymer electronics feel strain and evolve during operation
Advanced plastics play a key role in a range of emerging energy, computation, and biomedical technologies. However, understanding these complex materials under relevant device operating conditions poses a considerable challenge for conventional characterization techniques. In particular, understanding the dynamic aspects of these material systems under external stimuli is important for both improving material design and longevity.
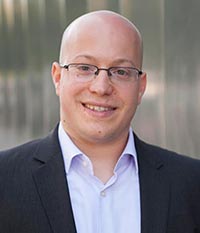
A new paper from a team including SQI member Jonathan Rivnay details how strain and dynamics couple with the external electrical stimulus within a complex polymer system. These systems have both electronic and ionic functionality and the insights developed enable future materials with enhanced device durability and performance in wet, operationally relevant conditions.
The group developed a technique allowing them to monitor the coupling of the structural deformation due to ions in a water-swelled polymer at levels below 0.1 percent with sub-second time resolution in response to external voltages. Rivnay and his colleagues discovered that the deformation induced by voltage and the resulting structural changes depend on how the voltage is applied to the material, whether through a large step or a gradual change. This finding, Rivnay said, is important because the deformation and structural changes are related to the material’s stability during electrochemical operation.
“As these materials are commonly used in bioelectronics, the improved understanding of their microscale structural changes pave the way for the creation of more stable devices of relevance for wearable and implantable technologies,” said Rivnay, Professor of Biomedical Engineering and Materials Science and Engineering at Northwestern and a co-corresponding author on the study. “The extended lifespan can lead to more reliable, sustainable, and long-lasting technologies reducing the frequency of replacements.”
Rivnay and his collaborators presented their work in the paper “Bridging Length Scales in Organic Mixed Ionic-Electronic Conductors through Internal Strain and Mesoscale Dynamics,” published February 26 in the journal Nature Materials. Ruiheng Wu, a postdoctoral researcher in Rivnay’s lab, was the paper’s lead author. Other contributors included co-corresponding author Christopher Takacs, an associate scientist at the SLAC National Accelerator Laboratory; most of the experiments were performed in Argonne National Laboratory with the help of coauthors Suresh Narayanan and Joe Strzalka.
The technique adapted by the researchers is also a novel way to understand the evolution of disordered electrically and ionically conducting polymer systems in the length scale of tens to hundreds of nanometers.
Using these experimental methods, the researchers found an asymmetry when disordered polymers change between conductive and non-conductive states. When transferring from conductive state to non-conductive state, the polymer switches very quickly. However, when transitioning from the non-conductive state to the conductive state, it takes significantly longer than expected to stabilize. This difference is due to the way complex electronic species rearrange. This discovery helps understand what is dictating the response time to devices like transistors and sensors, and opens a new possibility to use these unstable states to create new kinds of devices.
This work makes a significant contribution to understanding the structure-property relationship of organic mixed ionic-electronic conductors.
“The findings elucidate the complex coupling between charged species, microscale domain deformation, and external voltage,” Wu said. “This understanding is crucial for advancing our knowledge of device physics, particularly for materials designed to function in environments involving water.”
Except from the specific focus on the conjugated polymer system, these advances could also be a building block for future progress in general disordered systems.
“As the Advanced Photon Source upgrade nears completion, we expect the new light source will improve both time and spatial resolution of this technique,” Takacs said. “Harnessing this new source and the methodologies introduced in this work, we expect researchers to be able to explore a wide range of disordered systems, not just in response to voltage but also to other stimuli such as temperature and magnetic fields.”
Note: This article was first published by the McCormick School of Engineering.